Another form of nuclear energy is called fusion. Fusion means joining smaller nuclei (the plural of nucleus) to make a larger nucleus. The sun uses nuclear fusion of hydrogen atoms into helium atoms. This gives off heat and light and other radiation.
In the picture to the right, two types of hydrogen atoms, deuterium and tritium, combine to make a helium atom and an extra particle called a neutron.
Also given off in this fusion reaction is energy! Thanks to the University of California, Berkeley for the picture.
Scientists have been working on controlling nuclear fusion for a long time, trying to make a fusion reactor to produce electricity. But they have been having trouble learning how to control the reaction in a contained space.
What's better about nuclear fusion is that it creates less radioactive material than fission, and its supply of fuel can last longer than the sun.
Friday, June 11, 2010
Nuclear Fusion
Nuclear Fission
An atom's nucleus can be split apart. When this is done, a tremendous amount of energy is released. The energy is both heat and light energy. Einstein said that a very small amount of matter contains a very LARGE amount of energy. This energy, when let out slowly, can be harnessed to generate electricity. When it is let out all at once, it can make a tremendous explosion in an atomic bomb.

The word fission means to split apart. Inside the reactor of an atomic power plant, uranium atoms are split apart in a controlled chain reaction.
In a chain reaction, particles released by the splitting of the atom go off and strike other uranium atoms splitting those. Those particles given off split still other atoms in a chain reaction. In nuclear power plants, control rods are used to keep the splitting regulated so it doesn't go too fast.
If the reaction is not controlled, you could have an atomic bomb. But in atomic bombs, almost pure pieces of the element Uranium-235 or Plutonium, of a precise mass and shape, must be brought together and held together, with great force. These conditions are not present in a nuclear reactor.
The reaction also creates radioactive material. This material could hurt people if released, so it is kept in a solid form. The very strong concrete dome in the picture is designed to keep this material inside if an accident happens.
This chain reaction gives off heat energy. This heat energy is used to boil water in the core of the reactor. So, instead of burning a fuel, nuclear power plants use the chain reaction of atoms splitting to change the energy of atoms into heat energy.
This water from around the nuclear core is sent to another section of the power plant. Here, in the heat exchanger, it heats another set of pipes filled with water to make steam. The steam in this second set of pipes turns a turbine to generate electricity. Below is a cross section of the inside of a typical nuclear power plant.
Power plant drawing courtesy Nuclear Institute
Thursday, June 10, 2010
Heat Pipes
As the density of transistors in a microprocessor increases, the amount of heat disipated increases. A Pentium 4 processor (180 nm running at 2GHz) disipates, 55 Watts of power as heat. Its area is just 131 mm2. This gives a 55 W/(131/(102)) = 42 W cm-2. In comparison a steam iron is 5 Wcm-2.
One solution is the heat pipe. As its name suggests, it transfers heat from high temperature regions to lower temperature regions where there is more space for heat sinks or cooling fans.
Inside a heatpipe

The Phases of Matter
Matter can exist in several distinct forms which we call phases. We are all familiar with solids, liquids and gases. Whether a substance is a solid, liquid or gas depends on the potential energy in the atomic forces holding the particles together and the thermal energy of the particle motions. The pressure on the subtance also has an effect on the phase.
Solids
Crystaline Solids
Crystaline solids are characterised by a long-range order. The atoms are closely packed on lattice points held in in place by atomic bonds. The internal energy of the atoms is not sufficient to allow the atoms to break away from their lattice positions. Examples of crystaline solids include semiconductors, quartz, salt, etc.
Amorphous Solids
Amorphous Solids are still closely packed together but lack the translational symmetry of crystaline solids. However, even amorphous solids have relatively good spatial ordering, especially over small distances, (10-100 molecules)
Liquids
As the material is heated, the internal energy is increased and the atoms are no longer tied to their lattice positions but can move relative to each other although the atoms are still closely packed together.
Gases
A gas is matter in which the molecules are widely separated, move around freely, and move at high speeds. Examples of solids include the gases we breathe (nitrogen, oxygen, and others), the helium in balloons, and steam (water vapor).
The solid, liquid and gas phases of matter.

Eventually, given enough heat, the electrons and nucleus become separated and into positively, charged ions and negatively charged electrons. This soup of ions and electrons is known as a plasma
Phase Diagrams
The phases of the material can be recorded for many different pressures and temperatures. Plotting the phases, whether the material is solid, liquid or gas for many different pressures and temperatures we can build up a phase diagram for the substance.
Phase Diagram for water

Electric Current
Electric current is the flow of charge. The SI unit of current is the Amp. [A].
In mathematical terms we can describe the current as the rate of change of charge with time.
I = dQ/dt.
In order for the charge to flow there has to be a potential difference. V.
Microscopic Description
To produce a current the charge needs to flow in a conductor. This charge is carried by electrons. Consider a section of wire of length l and cross-section A, in which there is a potential difference. Electrons flow from the higher potential to the lower potential.
The total charge per unit volume = n
Therefore the total charge is dQ = nqAl, since Al is the volume of the wire and q is the charge on the electron.
If the electrons are moving at a speed vd. Then dt is l/vd seconds for the electrons to pass the point X.
The current I = dQ/dt = nqAvd
Free Electron Density
To calculate the number of electrons per unit volume in a metal we need to know some basic data such as the molar mass of the metal, density, the number of free electrons or valancy.
n = ρNA/M
If we know the current I and the number of electrons n we can calculate the drift-velocity. vd.
The drift-velocity is actually only around a millimeter per second.
For example, a current of 1 A at 6 V, how many electrons pass per second in a copper wire,
Conventional Current
One of the confussing aspects of current is the direction in which the current flows. It is an accident of history that the current was a flow of positive charge carriers. However, in metal conductors, the positive ions are not able to move and the flow of charge is a result of negative electrons. As a result the true direction of the current flow is in the opposite direction to the conventional current. It does not matter which is used as long as you are consistent.
To make it absolutely clear:

The electron flows from negative to positive.
Beyond the Solar System
Stars

Galaxies

Galaxies are large systems of stars and interstellar matter, typically containing several million to some trillion stars. The mass a galaxy is between several million and several trillion times that of our Sun. The distance across a galaxy can extension of a few thousands to several 100,000s light years. Typically, individual galaxies are separated by millions of light years distance. They come in a variety of shapes: Spiral, lenticular, elliptical and irregular. Besides simple stars, they typically contain various types of star clusters and nebulae.
Hubble classification of galaxies.

On a larger scale of the universe there are clusters of galaxies, superclusters
Exotic matter, black holes, quasars, pulsars
Radiation
Bubble Fusion
Sonoluminesence is the production of light from sound. It was first obversed in 1934, yet comparatively little is known about the process. Ultrasound in water can lead to the expansion and contraction of small bubbles dissolved in the water. In the reifaction, the bubble expands while during the compression, the bubbles collapse rapidly (1.4 km s-1 at the point of light emission) leading to a compression of the gas inside. The compression halts when the van der Vaals forces between molecules will not allow them to get any closer. The mechanism for the light emission is proposed by the shock-wave model.
The shock-wave model of sonoluminescence has the collapsing bubble generate an imploding shock-wave which focuses at a point. As the shock-wave propagates, it heats and intensifies the gas it passes through. At the focus, the shock-wave bounces back and makes the gas even hotter. Light is emitted because the shock-wave heats the gas enough to become ionised. The electrons emit light when they collide with the ions which results in the observed continuous emission spectra.
Experiments measuring the emission wavelength, show it to correspond to an energy of around 6 eV, which in turn, corresponds to a temperature inside a collapsing bubble of 70,000 K. There may be photons of even higher energy which would mean higher temperatures, but these wavelengths are absorbed by the water so it is not known what the maximum temperature inside the collapsing bubble is. It is at this point we turn our attention to fusion.
While 70,000 K is far below the 100 million K required for fusion, the temperature of the gas in the centre depends on the minimum size of the of the collapsing bubble. This is another unknown. The so-called shock radius, the radius of the bubble at the point of sonoluminescence, is around 0.1 μm but its minimum size could be smaller for an emission energy of 6 eV. If the radius of the bubble reaches 10 nm (just 10 times smaller) and the gas inside where deuterium, fusion could be ignited. Experiments producing sonoluminescence in deuterated acetone have claimed to produce nuclear fusion with the tell-tale emission of neutrons. Once again, the reproducibility of these results is at issue since it is very difficult to tell whether the neutrons were produced by nuclear processes or part of the background neutron count.
Cold Fusion
In 1989, an experiment performed at the University of Utah by Pons and Fleischmann's which claimed to have demonstrated fusion. The experiment was essentially an electrolysis cell, consisting of Platinum and Palladium electrodes placed in heavy-water (D2O). When the experiment was performed, it was found that the more heat was produced than can could be

The experiment hinges on the accuracy of the calorimetry used to determine the heating. More accurate calorimeters were tried and the heating effect was found to be significant. Other researchers found it difficult to reproduce the results so, after an initial media frenzy, the claims were widely dismissed.
Despite the general lack of acceptance by the mainstream scientific community, research continues by a few. In 2004, the Department of Energy peer reviewed the experimental evidence for cold fusion. It concluded that:
"The experimental data shows evidence of: the existence of a physical effect that produces heat in metal deuterides".
"The production of 42He in amounts commensurate with a 21H + 21H -> 42He reaction mechanism, a physical effect that results in the emission of energetic particles."
"The underlying process that produce these results are not manifestly evident from experiment. The scientific questions posed by these experiments are both worthy and capable of resolution by a dedicated program of scientific research".
Wednesday, June 9, 2010
Distances and Magnitudes
Distances
In the words of Douglas Adams, the author of The Hitch-Hiker's Guide to the Galaxy:
Space is big. You just won't believe how vastly, hugely, mind-bogglingly big it is. I mean, you may think it's a long way down the road to the chemist's, but that's just peanuts to space.
The distances involved in the universe are so vast that we must introduce some new units.
The Astronomical Unit A.U.
The most practical units we can use are related to the distance from the Sun to the Earth. This is the A.U. or Astronomical Unit. 1 Astronomical Unit = 149 598 000 km
The Parsec

How to calculate the parsec
Parallax is the apparent shift in the nearest stars due to the motion of the Earth around the Sun. The method of parallax gives rise to a natural distance unit that astronomers call the parsec (which we shall abbreviate as pc). The parsec is defined to be the distance at which a star would have a parallax angle p equal to one second of arc.

parsec in trigonometric terms.
Taking the unit is the parsec. This is defined as
1 Parsec = 3.08568025 × 1016 m. also used are kpc =1000 pc and Mpc =1 million pc
Light Year
The light year is the distance travelled by light in one year. All electromagnetic waves travel at a speed of x 299,792,458 ms-1 and an average year being 365.25 days. One light year is 299,792,458 x 108ms-1 x (365.25 x 24 x 60 x 60) s =
9.46073 x 1015 m. or 9.46073 x 1012 km.
With our new measuring sticks we can give a few examples of the scale of the universe.
The distance from the Earth to the nearest star after our Sun is 4.42 ly.
The Milky Way Galaxy is about 150,000 light-years across
The andromeda galaxy is 2.3 million light-years away.
The edge of the observable universe is 46.5 Giga light years away.
Tuesday, June 8, 2010
Moments and Couples
Moments and Couples
The moment of a Force or Torque
The moment of a force is the perdendicular force F acting at a distance, d and causes an object to rotate.
The moment of a force, also known as the torque T is defined as the force F acting at a perpendicular distance d.
T = F d(1)
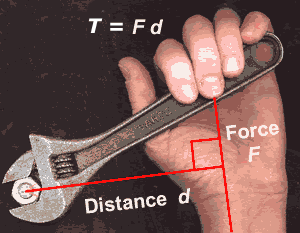
The dimension of moment is [M L2 T-2] which is the same as energy, however there is no direct relationship between the two. The SI unit of a moment is the Newton meter (Nm).
A special case of moments is where two anti-parallel forces separated by a distance d cause an object to rotate. This is known as a couple.
Couples
If two opposite moments act to cause an object to rotate, such as when your two hand are at the 'quarter-past-three' position on a car steering wheel, it is called a couple. The moment of a couple is called the torque. It is quite often said of engines and applys to the ability of the engine to turn the wheels, or wrongly by Jeremy Clarkson from 'Top Gear' as in, "This engine has a lot of torques."
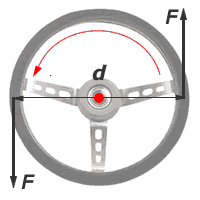
The torque is a psuedo-vector quantity. That is, it has magnitude and it has a direction that is projected perpendicular to the plane of the vectors F and r. The direction of the vector from the plane follows a right-handed corkscrew rule or equivalently the right-hand screw rule.
The magnitude of the torque of a couple can be found by finding the net torque from clockwise and anti clockwise moments acting on an arbitrary point of rotation.
T =F(x + d)
T =F x
Equating, F x +F d = F x.
Net Torque. T = F d
Newton's Laws of Motion
Newton's Laws of Motion
Newton's First Law
"A body in motion remains in motion unless it is acted on by an external force. If the body is at rest it remains at rest".
Newton's first law may seem counter intuitive to our everyday experience. If we move an object by pushing it, it will move and then come to a halt. To keep it moving at the same speed a force must be applied. However, most objects are slowed to a halt by friction or air-resistance. Newton's first law gives rise to the idea of inertia. It takes a large force to start to move an object with a large mass and it also takes a large force to stop it moving. A moving object has a reluctance to stop moving and a stationary object has a reluctance to move.
An external force is a force that is applied to the object. There may be other forces inside the object, for example, there are forces produced by the motion of the atoms that make up the object but on average, they cancel each other out and so do not effect the motion of the object.
Newton's first law applies in inertial reference frames, i.e. when the frame of reference is stationary or moving with constant velocity. It does not apply when the frame of reference is accelerating. Strictly speaking, the Earth is accelerating because it is rotating as well as orbiting the Sun, however the effect of this is small enough to consider the Earth as an inertial reference frame.
Newton's Second Law
"The force, F on an object is equal to the rate of change of momentum, p, with time".
In mathematical terms.
F = dpdt(1)
Or since p = mv, then
dpdt = d dt (mv) = m dvdt + vdmdt (using the product rule)
For constant mass, dm/dt = 0 is zero therefore,
F= mdvdt
We recognise dv/dt as the acceleration, a and so an alternative form of Newton's 2nd law is
F = ma(2)
and since, a = dvdt = d2rdt2
F = md2rdt2(3)
For this relationship to be true, we must measure the force F in Newtons [N], the mass m in kilograms and the acceleration in ms-2 otherwise there will be a constant of proportionality will have a value other than 1.
The force in Newton's 2nd law refers to the resultant force. If there are many forces acting on an object, it is the vector sum of those forces is the force that produces any acceleration.
∑Fext = ma(4)
Newton's Third Law of Motion
"Newton's third law applies to pairs of bodies. If a body A exerts a force on a body B, then body B exerts an equal and opposite force on body A".
Newton's third law reminds us that forces occur in equal and opposite pairs.
There are many examples of Newton's third law all around us. Every object that is on the floor or on top of another object is exerting a weight force toward the center of the Earth. If the object is stationary, then whatever the object is on top of is exerting an equal force in the opposite direction.
A rocket is also a good example of Newton's third law, it works by accelerating a mass in the form of hot gases, which produces a force. This produces an equal and opposite fore that pushes the rocket in the opposite direction.
The Importance of Newton's Laws
Newton's laws are extremely important not just in mechanics but in the whole of physics. When trying to understand a physical process, we often understand it by looking at the forces acting and working out the equations of motion. This is true of the motion of the planets to the flow of electrons in an electric or magnetic field.
Applications of Induction
Applications of Induction
AC Generator
The ac generator uses Faraday's laws of induction, it consists of a coil of wire rotating a magnetic field. As the coil rotates it cuts the magnetic flux generating an EMF, the EMF produced is given by Faraday's law. The angle is changing at the angular frequency ω. Therefore at a given moment in time the angle between the normal to the area and the magnetic field lines will be ωt. Therefore the flux linkage, φN will be BAN cos(ωt). Differentiating cos(ωt) function with respect to time gives -ω sin(ωt). Therefore, the EMF is given by
An AC Generator
The electrons flow first in one direction and then, in the other. The generator produces an alternating current. One advantage that AC has over DC is that it can easily be "stepped up" or "stepped down" with a transformer. In other words, a transformer can take a low-voltage current and make it a high-voltage current, and vice versa. Power is the product of voltage × current (P =VI). For a given amount of power, a low voltage requires a higher current and a higher voltage requires a lower current. Since metal conducting wires have a certain resistance, some power will be wasted as heat in the wires. This power loss is given by P = I2R. Thus, if the overall transmitted power is the same, and given the constraints of practical conductor sizes, low-voltage, high-current transmissions will suffer a much greater power loss than high-voltage, low-current ones. This holds whether DC or AC is used. However, it was very difficult to transform DC power to a high-voltage, low-current form efficiently, whereas with AC this can be done with a simple and efficient transformer.
Transformers
Another application for electromagnetic induction is the transformer. Transformers are used to either increase or decrease the magnitude of an alternating current. The structure of a transformer consists of two coils, called the primary and the secondary, linked by an iron core which is used to concentrate the magnetic field. When an alternating current is applied to the primary, it generates a magnetic field in the iron core the changing magnetic field induces an EMF in secondary coil. Depending on the ratio of turns in the primary coil to the secondary determines whether the transformer is a step-up or step-down.
Schematic diagram of a transformer
Spherical Convex Mirrors
Introduction of Spherical Convex Mirrors
Convex mirrors are also curved mirrors but the silvered surface bulges outward instead of inward as with the concave spherical mirror. As with all mirrors, the angle of incidence of a ray of light is equal to the angle of reflection with respect to a normal on the surface. On a curved surface the normal changes with the curvature.
Therefore, the light rays will diverge from the object. If the rays of light are extrapolated back to the point where they converge, this will be the point where the image is formed.
For reflections off a convex mirror, the image is virtual and upright.
Reflection off a convex spherical mirror
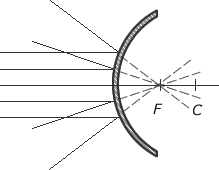
Use of Convex Mirrors
Convex mirrors are often used in car wing-mirrors because they have a greater field of view than a similar plane mirror. However the greater field of view is at the expense of making objects seem further away than they are in reality. Hence the expression seen etched on to the mirrors, 'Objects in Mirror are Closer than they Appear'. Concave mirrors are also used as security mirrors as they enable the viewer to see over a greater region of space.
Monday, June 7, 2010
Battery Operation
Why does this produce electricity? The flow of current can be understood as the flow of ions from the more reactice metal to the less reactive metal. The ions moving from one electrode to the other creates an electrical charge which is neutralised by the flow of electrons across the wire.
Before considering the reaction of two metals, consider what happens when we place a single metal electrode in an electrolyte. Some of the metal atoms in the electrolyte go into solution as ions while the remaining electrons create a negative charge on the metal. The separation of ions and electrons leads to a separation of charge. However, this build up of charge cannot continue indefinitely because as the negative charge builds up in the metal it becomes increasingly difficult for positive metal ions to go into solution. A similar build up in positive charge in the electrolyte also prevents the build up of charge. This degree of charge build up depends on the metal and represents the work required to separate electrons from the ions. This is known as the electro neutrality principle
Similarly, if a copper strip is placed in an aquaous Copper(II)Sulfate solution the copper will also lose ions. These reactions are often written as Cu | Cu+2 this is the half-cell reaction.
The tendancy for Zinc to lose ions is greater than that of Copper. When the two cells are joined together (using a copper wire to connect the electrodes and porous barrier that allows the ions to pass known as a salt-bridge connect the elecrolytes, the build up of electrons on the zinc will flow to through the wire onto the copper.
The copper ions in the electrolyte gain electrons and become copper atoms.
Thus the reaction can be written,
Zn | Zn2+ | | Cu2+ | Cu
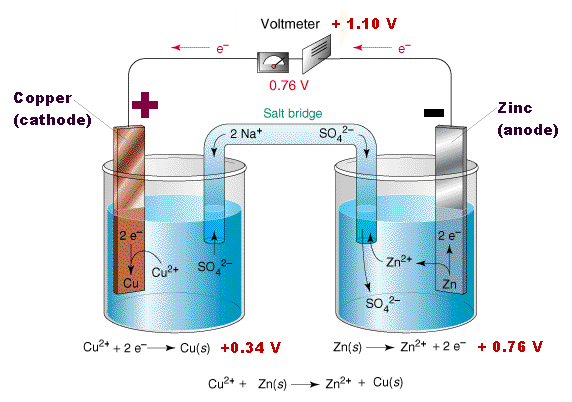
Electrical Cells and Batteries
Introduction
In it's most simple form a battery can be regarded as a pump that provides the energy to move charge around a circuit.
In order to provide a potential difference, or electro-motive force (EMF) a store of energy is required. One such method is a battery or cell. The common usages of the term battery is any device that converts chemical energy into electrical energy. However, strictly speaking, the term battery is used when several electrical cells are connected together to provide a source of a potential difference in a circuit. If it is just a single chemical source then it is called a cell.
History
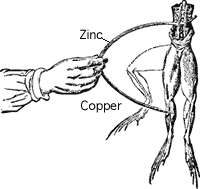
In 1791, Galvani noticed that a circuit created with two different metals, when touched on the ends of the leg of a dead frog, would cause it twitch. The two metals were creating an electric current within the frog's leg, causing the muscles to contract. Early batteries were an improvement of this method transferring chemical energy into electrical energy.
The first battery was invented in 1793 by Alessandro Volta. Just as the two different metals touching the wet skin of a frog's leg, caused an electrical current to flow, early batteries increased the voltage that could be produced by stacking a pile of discs made from silver and zinc sandwiched between paper soaked in a salt water solution as shown in Figure 2. In honour of Volta, we use the Volt as the unit of potential difference and EMF.